What are the different thermoplastic polymers used in microfluidics ?
Thermoplastics are highly crosslinked polymers that can be remolded multiple times after being cured by reaching glass transition temperature (Tg) [1] and that can retain their shape after cooling [2]. They are suitable for micro-machining processes but often unsuitable for long term cell cultures [1] due to their poor permeability to gas. Thermoplastics are generally optically clear, rigid, and resist to the permeation of small molecules.
There are two types of fabrication process that are broadly used for thermoplastics’ molding:
- thermomolding: a process requiring templates in metal or silicon for using at high temperature. It is excellent for commercial production (production of thousands of replicas at high rate and low cost) but not economical for prototypic use (due to the high cost of the engines that are required to perform thermomolding) [1].
- rapid prototyping using transfer molding (polydimethylsiloxane (PDMS) as replication intermediate). This technique is limited to thermoplastics with Tg’s below 150 °C because PDMS releases gas during molding at higher temperatures. It allow to transfer micropatterns to thermoplastics from easy-to-prepare photoresists [1]. BlackHole Lab offers 2 stations using the PDMS : the PDMS replication station and the PDMS membrane station.
The surface of thermoplastics can be modified depending on their applications: surface grafting and dynamic coating are two known methods [1]. These surface modifications are generally more stable than surface treatment on PDMS. For example, after treatment with oxygen plasma, thermoplastics surfaces can retain hydrophilicity for a few years. Unfortunately, conformal contact with other surfaces cannot be obtained with thermoplastics unlike PDMS [1].
In general, surface treatments performed by plasma, UV, or UV/ozone exposure can significantly improve bond strengths for thermoplastic microfluidics [3].
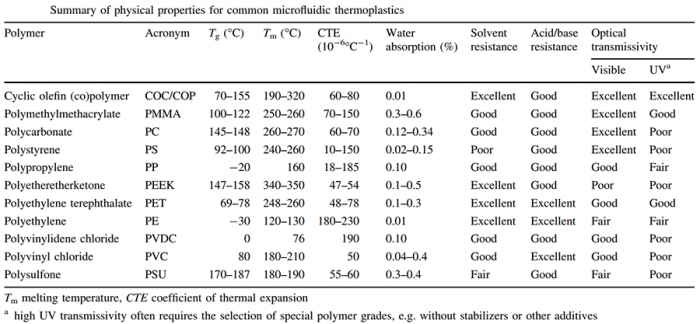
Important criteria to look at:
- permeability to gas: crucial for long-term cell culture in sealed microchannels but it comes with a high risk of creating bubble during microfabrication;
- optical transmissivity: crucial for observation;
- resistance to chemical products (acid, base, solvent…): important to avoid as much as possible the degradation of microchannels;
- resistance to swelling: critically important in nearly all soft lithographic techniques where a mold is brought into contact with a small amount of curable organic monomer or resin.
- hydrophobicity: hydrophobic surface of a material makes it prone to spontaneous nonspecific protein adsorption and cell adhesion when exposed to biological tissues or fluids.
I. Commonly used thermoplastics in microfluidics
I. 1. Polystyrene (PS) [4], [5]
Polystyrene (PS) is optically transparent, inert, biocompatible, rigid, cheap and is easily commercially available which makes it the most commonly used material in cell-culture. Its surface can be easily functionalized (various physical and chemical methods can be used including irradiation, corona-discharge or gas plasma) to make its hydrophobic surface more hydrophilic [1]. However, the requirement to use expensive equipment to realize complex chips from such a polymer (injection molding, hot embossing) [2] could be a major drawback for its use in a prototypic way, as PS is more adapted to mass manufacturing processes.
Multilayering of PS is possible in a short time (a few minutes) as it is molded and bonded quickly.
This polymer has become central to cell culture research and scientists are looking for potential use for PS other than cell bio-assays.
- Advantages: optically transparent, biocompatible, inert, its surface can be easily functionalized, adapted to mass manufacturing processes, commercial availability, interesting price, rapid bonding step.
- Drawbacks: expensive equipment required to realize complex chips from such a polymer, difficulties encountered in the thermal bonding step, more channels collapsing when width-to-height aspect ratios are too high.
- Common applications: cell culture research.
- Potential applications: cell culture on microfluidic chip (organ-on-chip) with either plasma treatment, or masking layer, or pre-coat the microchannels with extracellular matrix proteins prior to cell seeding, to enable cell adhesion and growth while also preventing bubble formation).
- Molding methods: injection molding, hot embossing.
- Bonding methods: thermal bonding, improvement of bonding strength with plasma treatment [3].
- Glass transition temperature: 92-107°C.
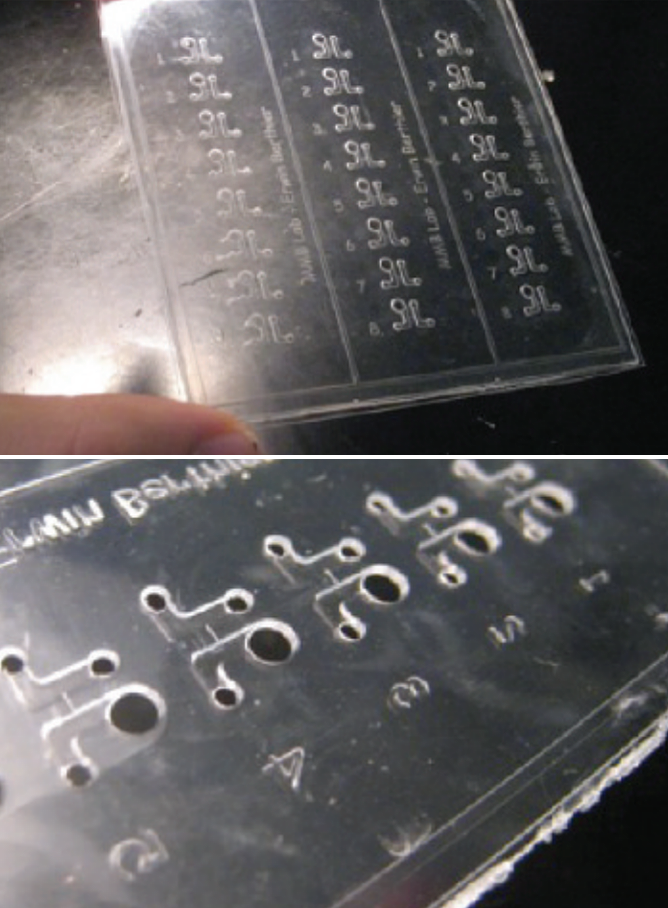
Rapid prototyping of arrayed microfluidic systems in polystyrene for cell-based assays. Young EW, Berthier E, Guckenberger DJ, Sackmann E, Lamers C, Meyvantsson I, Huttenlocher A, Beebe DJ.
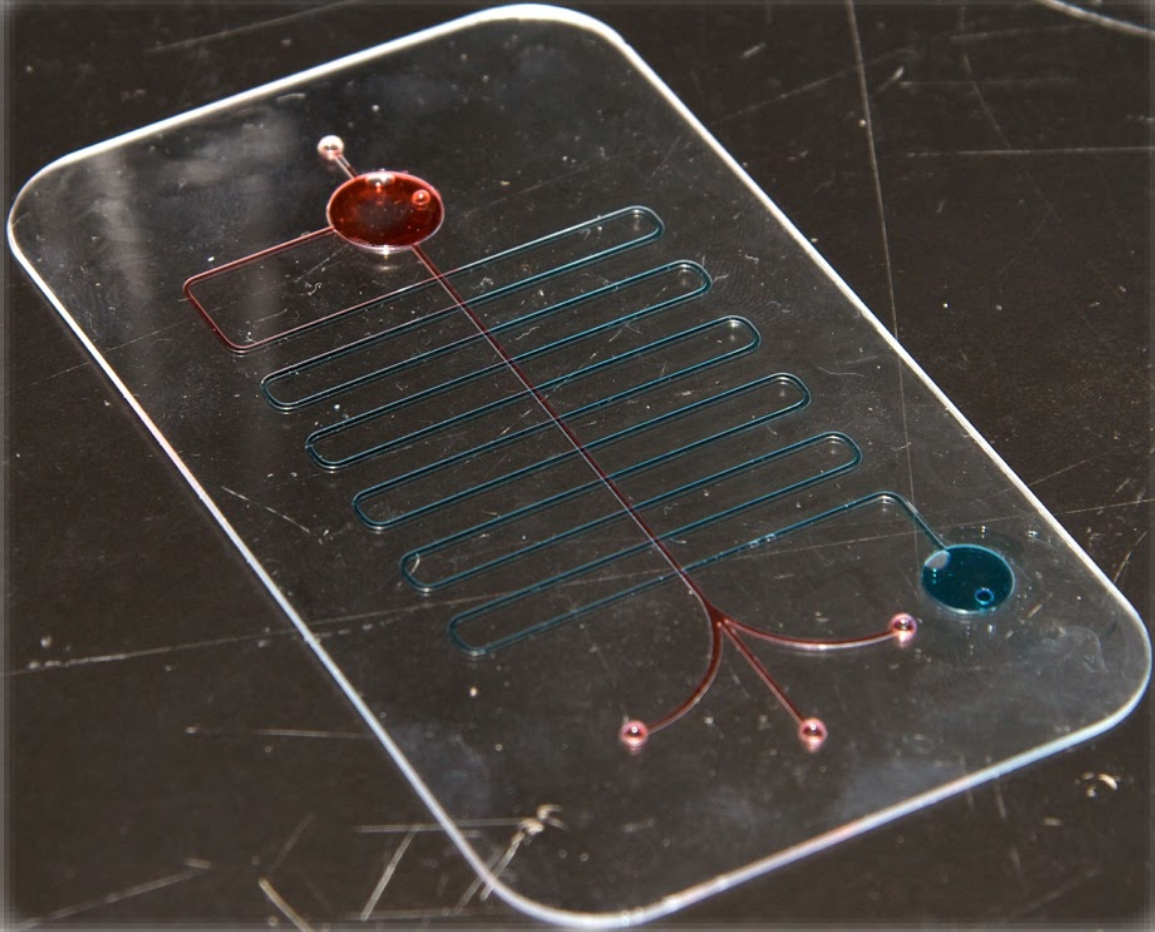
Fast-iteration prototyping and bonding of complex plastic microfluidic devices. Jonathan Siegrist, Mary Amasia, and Marc Madou.
I. 2. Polycarbonate (PC) [6], [7]
Polycarbonate (PC) is a durable material of choice for a range of microfluidic applications in biomedical studies and bioanalyses that include DNA thermal cycling applications like polymerase chain reaction (PCR) due to its transparency in the visible and its very high glass transition temperature (∼145°C). PC micro-features are also able to perform sample lysis, detection of pathogens, amplicon labeling, nucleic acid isolation, and enzymatic amplification [2]. This polymer also allows the fabrication of multilayer devices which makes PC a convenient alternative to protocols based on lithography and molding in PDMS.
But the fabrication of PC micro-features implies hot embossing technique and so induces subsequent annealing using thermal bonding. This bonding method is not a high quality bonding and has to be performed at high temperature for this polymer which might damage channel geometry.
- Advantages: durable material, transparency in the visible, very high glass transition temperature (∼145 °C), low cost, high impact resistance, low moisture absorption, good machining properties
- Drawbacks: poor resistance to certain organic solvents and absorbance in UV, quality and strength of bonding (thermal bonding only), alteration of the geometry of the channels due to bonding temperature.
- Common applications: DNA thermal cycling, fabrication of multilayer devices, enzymatic amplification, nucleic acid isolation, amplicon labeling, detection of pathogens, micro flow-injection amperometric determination of glucose.
- Molding methods: hot embossing with subsequent annealing of two layers using thermal bonding.
- Bonding methods: thermal bonding, improvement of bonding strength with plasma treatment [3].
- Glass transition temperature: 145-155°C.
I. 3. Poly(methyl methacrylate) PMMA [8], [9]
PMMA is a cheap easy-to-fabricate polymer which is the least hydrophobic one in the common plastic materials and which can be easily modified. Because of its low price, rigid mechanical properties, excellent optical transparency, and compatibility to electrophoresis, it is particularly useful for disposable microfluidic chips. It is also an ideal material for preparing ecological microchips which can be reused.
- Advantages: low price, least hydrophobic polymer in the common plastic materials, rigid mechanical property, excellent optical transparency, and compatibility to electrophoresis, ease of fabrication and modification, re-usable.
- Drawbacks: necessity of expensive equipment required to realize complex chips from such polymers (injection molding, hot embossing).
- Common applications: ecological microchips (re-usable), mixing analysis chip, DNA sequencer, electrophoresis chip.
- Molding methods: CO2-laser micromachining, injection molding, hot embossing, compression molding, and extrusion.
- Bonding methods: hot-press bonding (most common), microwave bonding, thermal fusion bonding and adhesive bonding, improvement of bonding strength with plasma treatment [3], using specific solvent conditions and sacrificial materials (ex: paraffin wax) can prevent the channels from collapsing.
- Glass transition temperature: 85-165°C (different grades).
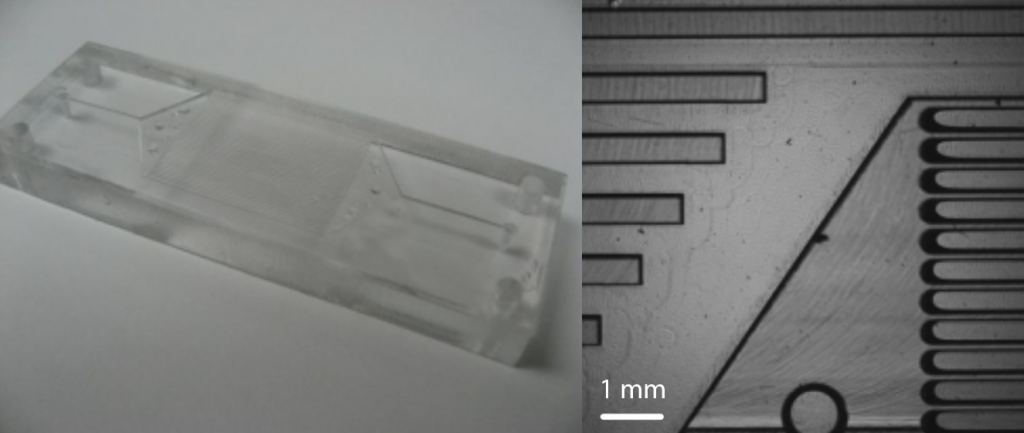
Fluorescence-based Sensing of 2,4,6-Trinitrotoluene (TNT) Using a Multi-channeled Poly(methyl methacrylate) (PMMA) Micro-immunosensor. Paul T. Charles, Andre A. Adams, Peter B. Howell, Jr., Scott A. Trammell, Jeffrey R. Deschamps, and Anne W. Kusterbeck.
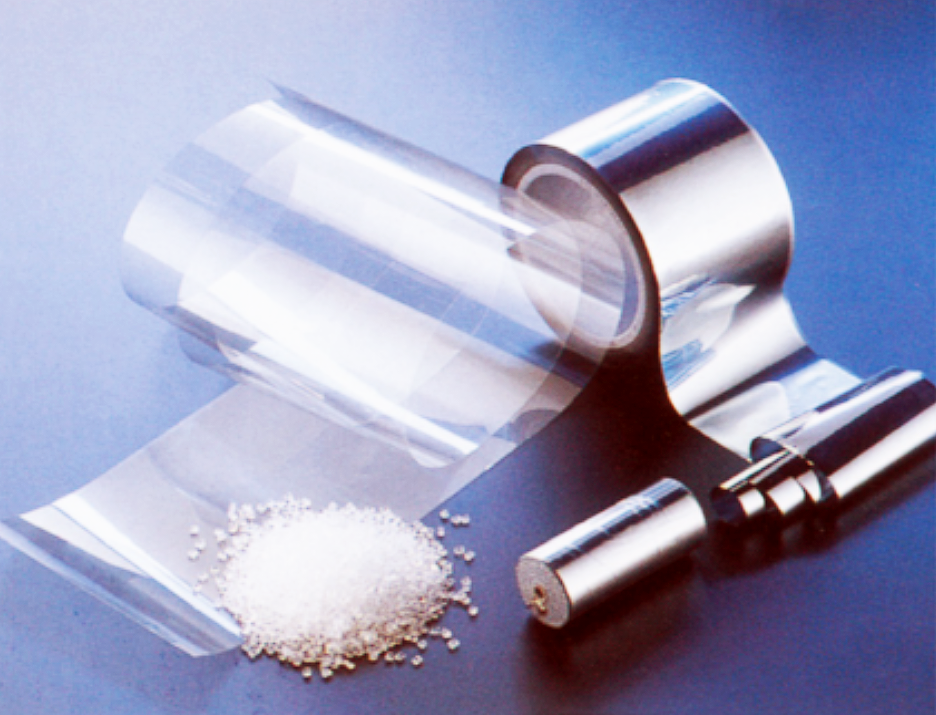
I. 4. Cyclic Olefin Copolymer (COC) [10]
Cyclic Olefin Copolymer (COC) is an amorphous thermoplastic copolymer consisting of ethylene and norbornene using a metallocene catalyst. This newer material has a wide variety of applications, including packaging films, lenses, vials, displays, and medical devices. Several types of commercial cyclic olefin copolymers based on different types of cyclic monomers and polymerization methods exist. The materials using a single type of monomer are more properly named cyclic olefin polymers (COP).
COC has good resistance to hydrolysis, acids, and alkalis and most organic polar solvents such as acetone, methanol, and isopropyl alcohol. COC material exhibit high transparency to light with wavelengths above 250 nm and has low autofluorescence. The transparency of COC to UV-light makes it an interesting material for lab-on-a-chip systems designed for bio-detection using integrated circuits. Devices made up of COC are not optimal for studies involving pharmaceuticals because of its hydrophobic surface, which makes it prone to spontaneous nonspecific protein adsorption and cell adhesion when exposed to biological tissues or fluids. In order to minimize adsorption of analytes such as proteins and to reduce the adhesion of cells, it is necessary to chemically modify the COC surfaces. Modification of COC surfaces has primarily been accomplished using photografting.
Solvent bonding has also emerged as an important method for sealing COC microchannels. Because COC is compatible with typical solvents used in high performance liquid chromatography (HPLC) such as acetonitrile, microfluidic systems based on COC are attractive for chip-HPLC applications [3].
- Advantages: good resistance to hydrolysis, acids, and bases and most organic polar solvents, high transparency to light with wavelengths above 250 nm, low autofluorescence, low birefringence, high Abbe number and high heat resistance, low water absorption, high dimensional stability with little change seen after processing
- Disadvantages: expensive equipment required to realize chips from such polymer (injection molding), hydrophobic surface, require surface treatment to minimize adsorption of analytes and to reduce the adhesion of cells.
- Common applications: packaging films, lenses, vials, displays, medical devices.
- Potential applications: lab-on-a-chip systems designed for bio-detection using integrated circuits.
- Molding methods: single and twin screw extrusion, injection molding, injection blow molding and stretch blow molding (ISBM), compression molding, extrusion coating, biaxial orientation, thermoforming and many others
- Bonding methods: solvent bonding (chip-HPLC applications), adhesives and thermal fusion bonding, improvement of bonding strength with plasma treatment.
- Glass transition temperature: 70-177°C (depending on the polymer content).
II. Less common thermoplastics in microfluidics – mainly used for valves
“The purpose of microvalves is to control flow within a microfluidics device, allowing them to become very complex and more automated. Without microvalves, all reactions and mixing must occur in the same space, unless they were premixed elsewhere, which might just eliminate the advantage of microfluidics.” (Source).
A very complete review about microvalves has been written by Kwang W Oh and Chong H Ahn (Journal of Micromechanics and Microengineering) “A review of microvalves”.
II. 1. Poly(ethylene glycol) diacrylate (PEGDA) [12]
Poly(ethylene glycol) diacrylate (PEGDA) is a polymer which is stable in water, optically transparent, biologically inert, has good and variable mechanical properties and has low background fluorescence. However, it has greater resistance to permeation of small hydrophobic molecules and shows less nonspecific adsorption than PDMS. Moreover, polymerized PEGDA (Poly-PEGDA) is not immunogen, resistant to protein adsorption, however, it does not permit cell adhesion. PEGDA is a convenient material for microfluidics since it does not require too much energy and its polymerization can occur rapidly at room temperature.
- Advantages: water stability, optical clarity, low background fluorescence, shows less nonspecific adsorption and has greater resistance to permeation of small hydrophobic molecules than PDMS, biologically inert
- Common applications: PEGDA: tissue engineering (scaffolds); Poly-PEGDA: robust microfluidic valves and pumps in different shapes for application in small volume assays, strong potential for application in latch-valve designs (elastic modulus [∼0.1 GPa]) [2].
- Cannot be used for: self-collapsing valves (elastic modulus [∼0.1 GPa]) [2].
- Molding methods: Serial replica molding (PDMS support), casting (Poly-PEGDA).
II. 2. Teflons: perfluorinated compounds (FEP/ PFA/PFPE/PTFE) [2], [13]
The fabrication and use of whole-Teflon chips in microfluidics is quite recent and presents as its main advantage to be low-cost and fast. Teflons present non-sticky and outstanding antifouling properties, are optically transparent, soft enough to make diaphragm valves, and moderately permeable to gases [1] (cells can be cultured in-channel for extended periods of time), oleophobic, hydrophobic, show cellular compatibility over 5 days, have a good gas permeability, have low nonspecific protein adsorption compared with PDMS and PS and are extremely inert to chemicals and solvents. Teflons have a high melting point (> 280 °C) which means that complex microstructures can be fabricated by high-temperature thermo-molding with nanometer resolution. Moreover, micro-structured devices made of perfluorinated compounds maintain their elastomeric properties up to 200°C.
- Advantages: extremely inert to chemicals and solvents, non-sticky and antifouling properties, optically transparent, soft enough to make diaphragm valves, moderately permeable to gases, low nonspecific protein adsorption compared with PDMS and PS.
- Common application: non-stick coatings and in superclean process, superior for applications involving corrosive chemicals, strong solvents, high and low temperatures, and pressurized processes.
- Possible molding method: hot-embossing with a template (adapted from soft-lithography technique) [13].
- Possible bonding method: thermobonding coupled with holding scaffold (stainless steel screw clamps) [13].
II. 2. 1. Fluorinated ethylenepropylene (FEP) and Perfluoroalkoxy (PFA)
Perfluoroalkoxy (Teflon PFA) and fluorinated ethylene propylene (Teflon FEP), are perfluorinated polymers that are used to build microfluidic devices. They are thermo-processable and can be thermally bonded to other material to create composites (ex: glass−FEP used to build latch-valve devices), which are resistant to chemical solutions that are highly corrosive.
PFA is a slightly opaque perfluorinated compound so fluorescence and cellular imaging can still be used.
- Specific advantages: resistance to organic solvents, resistance to corrosion and stress-cracking, as well as to molecular adsorption and molecule leaching from the polymer bulk to solutions [14], extreme chemical capability and robustness over a broad range of temperature from -100°C to 220°C (PFA), can be directly re-used for another thermomolding process without leaving bubbles inside (PFA), anti-fouling properties (PFA).
- Specific drawbacks: slightly opaque compound (PFA), requires high-temperature hot embossing (∼260 °C) to mold devices (PFA), the bonding to glass and similar substrates tends to be very weak.
- Common applications: latch-valve devices.
- Potential applications: most promising materials for the fabrication of cheap, solvent resistant and reusable microfluidic chips (can be recycled for multiple uses without the risk of contamination).
- Molding methods: high-temperature thermomolding.
- Bonding methods: melt-processability by conventional thermoplastic processing methods.
- Glass transition temperature: PFA – 90°C.
II. 2. 2. Polyfluoropolyether diol methacrylate (PFPE-DMA) [15]
Some microfluidic devices use polyfluoropolyether (PFPE) or polyfluoropolyether diol methacrylate (PFPE-DMA) because of its numerous attractive properties. PFPEs are fluoropolymers that are liquids at room temperature prior to curing, exhibit low surface energy, low modulus, high gas permeability, is compatible with all solvents involved in DNA synthesis reactions and have a low toxicity with the added feature of being extremely chemically resistant like Teflon. Moreover, PFPE channels shows no evidence of swelling [15].
The main advantage of this polymer is its photocuring ability which could extend the use of microfluidics devices to new chemical applications [15]. With this characteristic, the production time of microfluidic devices can be reduced from several hours to a matter of minutes.
- Specific advantages: low surface energy, high durability and toughness, high gas permeability, low toxicity, photocuring ability (reduces the time of production), molding with resolutions as small as 50nm [16], PFPE-DMA showed less swelling in the presence of organic solvents than PDMS [17].
- Specific disadvantages: multilayering: bonding separate layers can be problematic [12].
- Applications: valves, microchannels with integrated valves [15].
- Molding methods: serial replica molding (PDMS support), casting, spin-coating.
- Bonding methods: bonded strongly to PDMS with UV-curing.
II. 2. 3. Polytetrafluoroethylene (PTFE) [20]
PTFE Teflon® Tubing (PolyTetraFluoroEthylene, often described as Teflon® tubing) is a transparent, chemically inert and non-toxic material that features unmatched chemical resistance and a surface that really facilitates the flow. Due to its high flexibility, PTFE is mostly used with microfluidic fittings in low-pressure applications. Its melting point is reached at 327°C, making it a material of choice in high temperature applications.
- Specific advantages: high flexibility, resistance to chemical corrosion, non-absorption of moisture, chemically inert and non-toxic material, very high melting point.
- Specific disadvantages: non-uniform features below 200 μm.
- Common applications: low-pressure and high temperature applications, inkjet and biomedical analysis devices, tubing.
- Bonding methods: isostatic or compression bonding.
- Glass transition temperature: 130°C.
III. Anecdotal thermoplastics for microfluidic chips
III. 1. Polyurethane (TPU) [18], [19]
Thermoplastic polyurethanes (TPU) are a class of polyurethane plastics and are technically thermoplastic elastomers consisting of linear segmented block copolymers composed of hard and soft segments. They are characterized by their high-mechanical strength, biocompatibility, resiliency, and good resistance to abrasion. However, TPU present hydrophobic surfaces that are water repellent and protein adsorbent. But, with surface treatment to make them more hydrophilic, their utility can be improved. To limit those phenomena and improve the utility of these polymers, modification of their surface (with hydrophilic polymers, photo-chemical reactions or various grafting techniques) has been demonstrated on open surfaces or films of TPU. Surface modification inside the microchannels is a different challenge: for example, rapid depletion of reagents due to high surface to volume ratio has to be handled.
- Advantages: high-mechanical strength, resiliency, good resistance to abrasion.
- Drawback: rigid, opaque, difficult to connect with tubing, hydrophobic surfaces, non-specific adsorption (can be improved by surface modification), not suited for rapid prototyping, expensive microfabrication process (equipment and intermediates moulds).
- Common applications: artificial heart, intra-aortic balloons, pacemaker leads, heart valves, hemodialysis membranes. Microfluidics: microvalves.
- Molding methods: rotating plate (solvent molding) for TPU films and sheets, vertical dipping and rotating mandrel (solvent molding) for fabricating cylindrical parts, like tubing. For replicating microscale features, injection moulding, imprinting, plasma etching, sacrificial material and reaction polymerization are used.
III. 2. Polyetheretherketone (PEEK) [20]
This biocompatible and very chemically compatible material is widely described as the highest performing thermoplastic material currently available because of its remarkable chemical resistance. However, due to a swelling effect, take caution when using methylene chloride, THF, and DMSO. Nevertheless, these qualities make PEEK tubing and capillaries a material of choice in numerous low and high pressure applications.
- Advantages: chemical resistance, biocompatibility.
- Drawback: swelling risk with methylene chloride, THF, and DMSO.
- Common applications: valves, tubing, capillaries.
- Glass transition temperature: 147-158°C (up to 250°C for a few grades).
III. 3. Polyethylene terephthalate (PET) and polyvinyl chloride (PVC)[3]
Both of these materials have been mentioned as microfluidic reference but the publications about them are rare. So there is not so much information about their advantages, drawbacks, microfabrication processes, etc.
PET presents the advantages of having a low glass transition temperature (67-81°C) and to be really resistant to solvent, acid and base. It is molded by hot embossing method and is bonded with thermal bonding (the bonding strength can be improved with plasma treatment).
Concerning PVC, it is known that it can be molded by imprinting, laser ablation or hot embossing. It has a low glass transition temperature (80°C), a good optical transmissivity for visible light and a good resistance to solvent, acid and base. However, it has a poor transmissivity for UV light.
References
[1] Ren, K.; Zhou, J.; Wu, H., Materials for Microfluidic Chip Fabrication. Acc. Chem. Res., 2013, 46 (11), pp 2396–2406.
[2] Nge, P. N.; Rogers, C. I.; Woolley, A. T., Advances in Microfluidic Materials, Functions, Integration, and Applications, Chem. Rev., 2013, 113 (4), pp 2550–2583.
[3] Tsao, C.-W.; DeVoe, D. L., Bonding of thermoplastic polymer microfluidics, Microfluidics and Nanofluidics. 6(1):1-16 · January 2009.
[4] Chen, C. S.; Breslauer, D. N.; Luna, J. I.; Grimes, A.; Chin, W. C.; Leeb, L. P.; Khine, M., Shrinky-Dink Microfluidics: 3D Polystyrene Chips. Lab Chip, 2008, 8, 622–624.
[5] Young, E. W. K.; Berthier, E.; Guckenberger, D. J.; Sackmann, E.; Lamers, C.; Meyvantsson, I.; Huttenlocher, A.; Beebe, D. J., Rapid Prototyping of Arrayed Microfluidic Systems in Polystyrene for Cell-Based Assays. Anal. Chem., 2011, 83 (4), pp 1408–1417.
[6] Ogończyk, D.; Węgrzyn, J.; Jankowski, P.; Dąbrowski, B.; Garstecki, P., Bonding of Microfluidic Devices Fabricated in Polycarbonate. Lab Chip, 2010,10, 1324-1327
[7] Wang, Y.; He, Q.; Dong, Y.; Chen, H., In-Channel Modification of Biosensor Electrodes Integrated on a Polycarbonate Microfluidic Chip for Micro Flow-Injection. Sensors and Actuators B 145 (2010) 553–560.
[8] Zhang W.; Lin S.; Wang C.; Hu J.; Li C.; Zhuang Z.; Zhou Y.; Mathies R. A.; Yang CJ., PMMA/PDMS Valves and Pumps for Disposable Microfluidics. Lab Chip. 2009 Nov 7;9(21):3088-94
[9] Chen Y.; Zhang L.; Chen G., Fabrication, Modification, and Application of Poly(methyl Methacrylate) Microfluidic Chips. Electrophoresis. 2008 May;29(9):1801-14.
[10] Jena, R. K.; Yuea, C. Y., Cyclic olefin copolymer based microfluidic devices for biochip applications: Ultraviolet surface grafting using 2-methacryloyloxyethyl phosphorylcholine. Biomicrofluidics. 2012 Mar; 6(1): 012822–012822-12.
[12] Rogers, C. I.; Pagaduan, J. V.; Nordin, G. P.; Woolley, A. T., Single-Monomer Formulation of Polymerized Polyethylene Glycol Diacrylate as a Nonadsorptive Material for Microfluidics. Anal. Chem., 2011, 83 (16), pp 6418–6425.
[13] Ren, K.; Dai, W.; Zhou, J.; Su, J.; Wu, H., Whole-Teflon microfluidic chips. 2011. PNAS, vol. 108, no. 20. 8162–8166.
[14] Stojkovič, G.; Krivec, M.; Vesel, A.; Marinšek, M.; Žnidaršič-Plazl, P., Surface Cell Immobilization within Perfluoroalkoxy Microchannels. 2014. Applied Surface Science 320:810-817.
[15] Van Dam, R. Michael, Solvent-resistant elastomeric microfluidic devices and applications. 2006. California Institute of Technology. Chapter 5. 98-122.
[16] Rolland, J. P.; Hagberg, E. C.; Denison, G. M.; Carter, K. R.; De Simone, J. M., High-Resolution Soft Lithography: Enabling Materials for Nanotechnologies, Angew. Chem. Int. Ed. 2004, 43, 5796 –5799.
[17] Rolland, J. P., Van Dam, R. M.; Schorzman, D. A.; Quake, S. R.; DeSimone, J. M., Solvent-Resistant Photocurable “Liquid Teflon” for Microfluidic Device Fabrication, J Am Chem Soc. 2004;126:2322.
[18] Piccin E.; Coltro WK.; Fracassi da Silva JA.; Neto SC.; Mazo LH.; Carrilho E., Polyurethane from Biosource as a New Material for Fabrication of Microfluidic Devices by Rapid Prototyping. J Chromatogr A. 2007 Nov 30;1173(1-2):151-8.
[19] Wu WI.; Sask KN.; Brash JL.; Selvaganapathy PR., Polyurethane-Based Microfluidic Devices for Blood Contacting Applications. Lab Chip. 2012 Mar 7;12(5):960-70.
For general information
For further information, do not hesitate to contact our team of experts:
contact@blackholelab.com or phone: +33(0).782.831.207